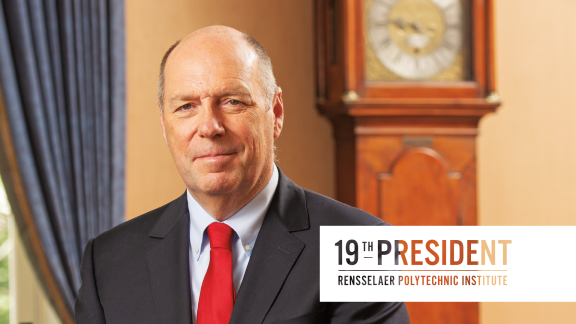
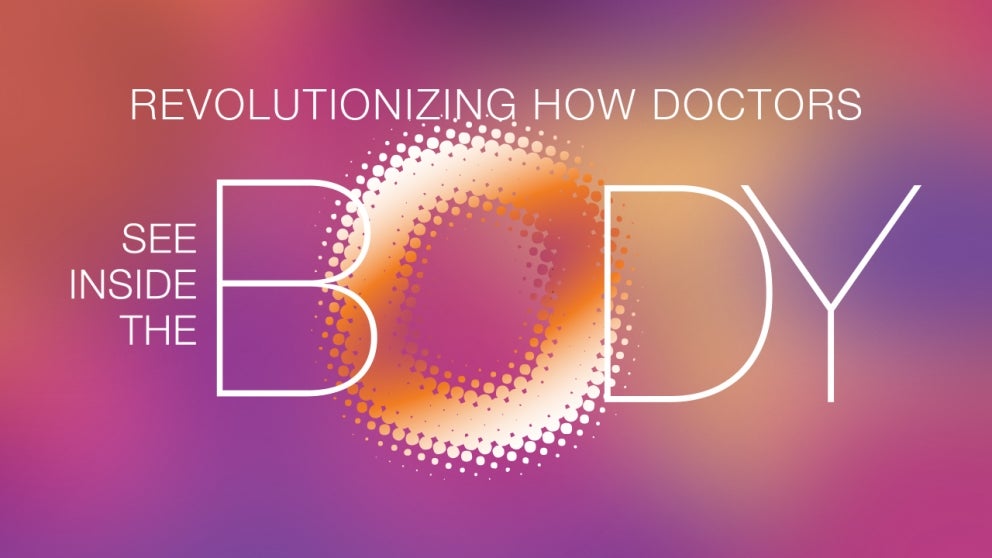
Revolutionizing How Doctors See Inside the Body
Artificial intelligence and deep learning are enabling a more accurate view of patients.
By Torie Wells
On the ground floor of the Center for Biotechnology and Interdisciplinary Studies (CBIS) is a brightly lit lab, broken into two rooms, bisected by a wall with a small glass window. On one side of the window is a set of large computer monitors that can easily pull up detailed images of organs and skeletal structures. On the other is a space full of X-ray machines feeding information through a multitude of wires.
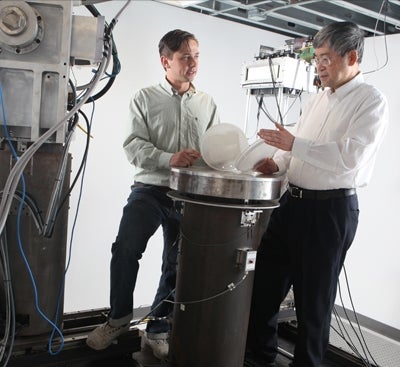
Ge Wang, an expert in biomedical imaging at Rensselaer, stands next to a massive metal imaging machine as he speaks; it’s taller than he is and takes up about a third of the lab. This universal X-ray imaging benchtop system, he explains, can imitate any medical computerized tomography (CT) scanner — enabling quite a bit of his research.
The setup evokes images of a medical facility, which is quite fitting, because inside this lab engineers are confronting one of the most persistent health challenges humanity currently faces: cancer.
“We are helping medical doctors do their job better,” says Wang, the Clark and Crossan Endowed Chair Professor of Biomedical Engineering and director of the Biomedical Imaging Center within CBIS. “Imaging is the physician’s and doctor’s eyes.”
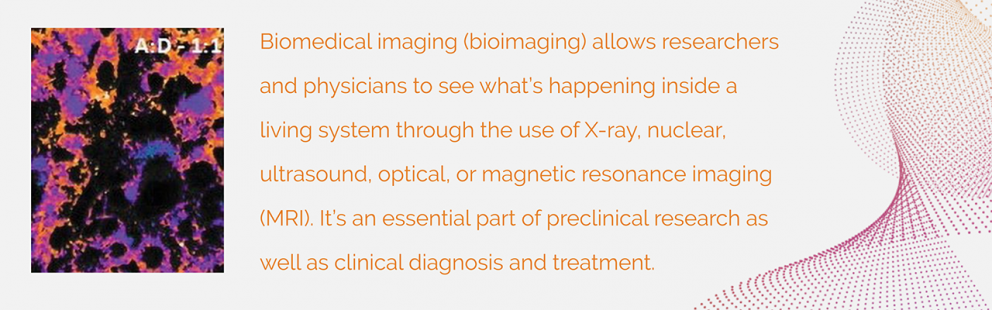
If technologies like Wang’s X-ray machine are the “eyes” of the imaging field, then researchers at Rensselaer could be considered the “brain.” Collaborative teams are developing the algorithms, computational techniques, and even new technologies that are needed to make humanity’s look inside the body clearer, more detailed, and more useful. These researchers are tackling the challenges of bioimaging from different angles, but as they begin to combine their knowledge, the strides they are making to advance the field are accelerating.
In the past five years, bioimaging research projects led by biomedical engineering faculty have received more than $16.5 million in federal grants, says Juergen Hahn, head of the Department of Biomedical Engineering — a total that says something about the importance of this work, and the expertise Rensselaer has to offer.
“X-rays have been used in medicine for over a hundred years. However, the coupling of new technologies with powerful computing and AI-based algorithms at Rensselaer is making our vision into the human body far clearer, enabling better and faster diagnoses and treatments,” says Shekhar Garde, dean of the School of Engineering. “Large federal grants, outstanding papers in top journals, and awards from professional societies all suggest that we have built a very strong team in this area.”
Many of the projects that researchers are working on sound much like science fiction, but their implications could have very tangible effects on human health. If doctors, for instance, could observe drug delivery and its effect on cancer cells in real time, they could tailor treatment with unprecedented specificity.
One example, a multidisciplinary, academic-industrial research project — led by Wang, Xavier Intes, professor of biomedical engineering, and Margarida Barroso, who is with Albany Medical Center — aims to make that possible for the study of breast cancer, in collaboration with MARS Bioimaging Ltd. The researchers are combining X-ray and optical imaging technologies for preclinical research, with the support of a nearly $2.9 million grant from the National Institutes of Health (NIH).
Another $3.1 million National Cancer Institute-funded project led by Intes, Barroso, and David Corr, associate professor of biomedical engineering, is combining advanced optical imaging techniques with bioprinting to explain the fundamental mechanisms that prevent drugs from interacting with cells.
“Cancer is not static. Cancer is dynamic. When you start to introduce drugs, the disease can change rapidly, so noninvasive imaging is important,” says Intes. “We are combining cutting-edge technologies that we had to develop ourselves at RPI to enable the potential of precision medicine.”
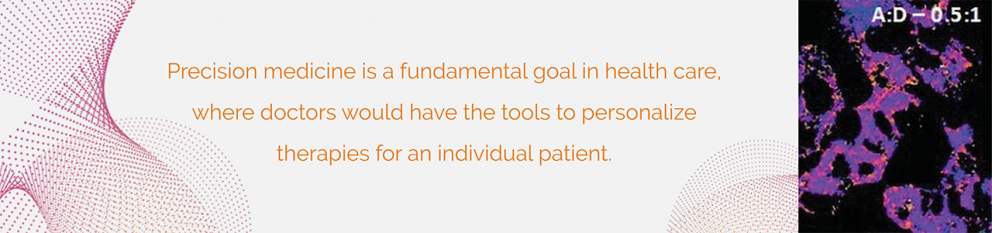
While these projects are for in vitro and preclinical research, so humanity can better understand diseases and how to treat them, other Rensselaer research is quickly moving toward use in a clinical setting.
“Imaging has become an essential component of modern medicine,” says Pingkun Yan, assistant professor of biomedical engineering, who is partnering with Wang and Intes on several research initiatives while also leading his own.
Yan is currently developing an ultrasound imaging method that will help radiation oncologists accurately differentiate between a prostate tumor and healthy tissue, so they can accurately deliver radiation to the cancer cells. This research is also being supported by NIH.
“If you use imaging methods together with other advanced image analysis techniques, you can actually better customize a treatment for a specific patient,” Yan says.
Beyond bioimaging, what unites the researchers’ work is their expertise in machine learning, especially deep learning. The advancement of artificial intelligence (AI) is enabling the production of clearer, more accurate images — often more quickly and with a lower radiation dose or light damage.
For example, Intes and Yan recently showed how a deep neural network, which they developed, could improve molecular-level imaging of interactions within a cell — a necessary tool when trying to identify disease and evaluate the effectiveness of drugs. They published their findings in Proceedings of the National Academy of Sciences.
In other research, recently published in Nature Machine Intelligence, Wang’s team, in collaboration with Massachusetts General Hospital investigators, demonstrated how artificial intelligence can improve low-dose CT images. This is an important step in the constant pursuit to reduce radiation exposure for patients.
“We are exploring great opportunities now,” Wang says. “Artificial intelligence and deep learning technology will have a revolutionary impact on medical imaging. It’s not just another technology; we view this as a paradigm shift.”
The newest supercomputer at Rensselaer, AiMOS, which went online late last year, is helping to enable some of the immense data processing and number crunching that’s necessary for these advancements.
“The confluence of AI and biomedical imaging is expanding horizons in multiple dimensions,” says Suvranu De, the J. Erik Jonsson ’22 Distinguished Professor of Engineering, and co-director of the Center for Modeling, Simulation, and Imaging in Medicine (CeMSIM) with Intes.
Using noninvasive optical brain imaging, De and Intes have shown they can evaluate which areas of the brain are active when a novice or expert surgeon is performing surgical tasks both in real and virtual training environments.
Lasers measure which parts of the brain are most active, indicating when someone is learning and when a response has been mastered. The goal is to improve surgeon training and certification processes. The researchers are working closely with key stakeholders such as the Society of American Gastrointestinal and Endoscopic Surgeons and the American College of Surgeons.
“We are developing tools that are providing unique insights in outstanding clinical questions but also that are going to be far more amenable for the end-users, meaning the biologists, neuroscientists, but also the surgeons,” says Intes.
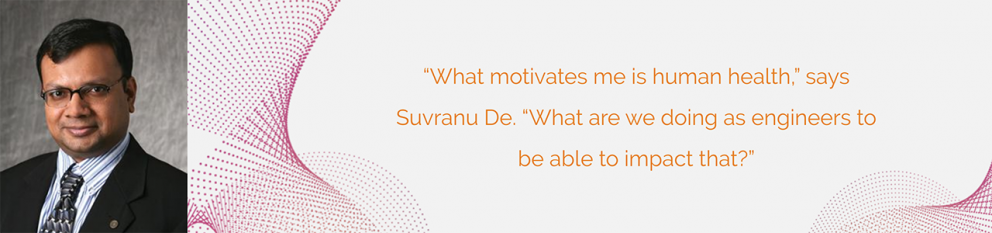
The team is expanding this research, De says, to include a deep neural network — developed by Intes and Yan — that predicts the scores a surgeon would earn during board certification, just by evaluating brain signals. The researchers are also working on computer vision algorithms to add visual confirmation.
Using ultrasound waves, De says, researchers in CeMSIM are combining imaging with biomechanics. By assessing the mechanical properties of soft biological tissues, they can determine how bad and how deep a burn is. Raman spectroscopy — a type of electromagnetic radiation that measures vibrational and rotational movement among molecules — is adding additional information about protein changes within the tissue. These research efforts involve collaborations with leading clinical institutions like Harvard Medical School, Mount Sinai Hospital, and the University of Washington.
At its core, Garde says, this success is a result of the convergence of different disciplines. The researchers bring strong skills in mathematics, advanced computing, and algorithms, and apply them to important problems in life sciences and human health.
“If you want to fight cancer, then math, computing, and engineering must be part of your toolbox, along with the knowledge of cancer biology,” Garde says.
Back inside his lab, Wang shows some of the progression he’s seen in just a few years, beginning with the universal CT scanner that he’s standing next to.
The device develops images in grayscale, which is what most people are familiar with, but Wang and his team are also working to improve another technology that develops images in color. That improvement will help researchers and doctors distinguish between different tissues, bone, muscle, and contrast agents. Wang believes it will be as widely used as grayscale X-ray medical imaging within 10 years.
His lab is also working on a device that, by measuring X-ray refraction and scattering, would tell doctors enough critical information about tumor tissue to know if it’s benign or malignant. That, he believes, is the future of imaging.
Whichever innovation is next, Wang already has the satisfaction of knowing that his research has had a global impact. Early in his career, he developed and published the first helical/spiral cone-beam/multi-slice CT algorithm — a type of CT imaging method that has benefited patients in hospitals worldwide, even his own mother.
“That is a really good feeling,” he says with a smile. “It’s having scientific curiosity and meeting technical challenges that make you feel greatly satisfied. That, and serving people across the world.”