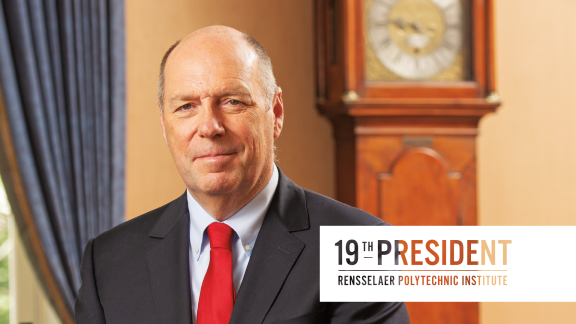
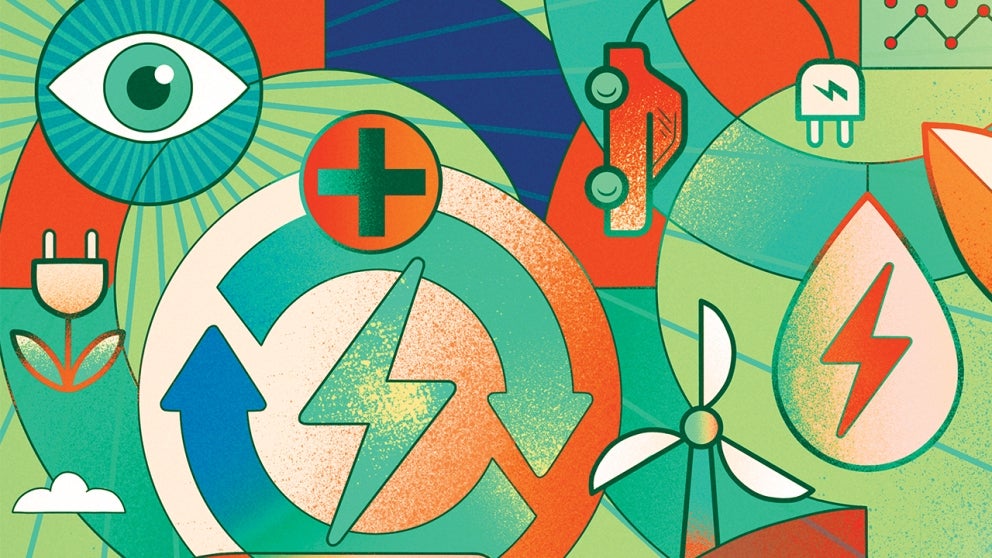
Renewable Revolution
Through his work on transforming lithium-ion batteries, Nikhil Koratkar is revolutionizing the way we view energy storage.
By Torie Wells
On any given day, Nikhil Koratkar and his research team may be pouring over spectroscopy data, or analyzing battery chemistry results, or evaluating graphs. Much of their time in the lab is spent trying to decipher what the data tells them. On any given day, they may also notice something unusual, something unexpected in their findings. And on those days, Koratkar says, everything shifts.
We drop everything else and focus like a laser beam on that specific discovery,” he says. “This phase is by far the most exciting in the lab, when we know we have something important to share with the community.”
For the past 10 years, Koratkar, the John A. Clark and Edward T. Crossan Chair Professor of Mechanical Engineering, has devoted his research to understanding how the lithium-ion batteries we currently use in our laptops, cell phones, and emerging solar and electric vehicle technologies can be made safer, more efficient, less expensive, and greener. He and his team model and simulate the performance of new materials and configurations, and then build small batteries, such as coin or pouch cells, to test those theories.
From each data point to experiment, a quiet current of urgency flows as the world’s need for energy storage rapidly changes.
“There’s no question that as the size of the population keeps growing and as society becomes increasingly advanced, we need more and more energy,” Koratkar says. “The global requirement for energy on the planet is going to keep increasing exponentially and to meet this, batteries are going to play a crucial role.”
He spoke about his research over Webex on a Friday afternoon. The battery in his laptop became all the more critical when the world rapidly shifted to remote work in the face of a global pandemic. But, Koratkar doesn’t see computers and cell phones as the biggest driver behind the world’s future energy storage needs. Instead, he sees the largest growth potential in batteries that will allow society to reduce its carbon footprint: energy storage for solar and wind power, and electric vehicles.
“Besides performance, cost and safety are pivotal for transportation and grid storage batteries,” Koratkar says. “The cost of a lithium-ion battery is too expensive; it needs to come down. At the same time, safety is extremely important. If these batteries are unsafe, and they are being deployed on a massive scale across the globe, that’s a problem.”
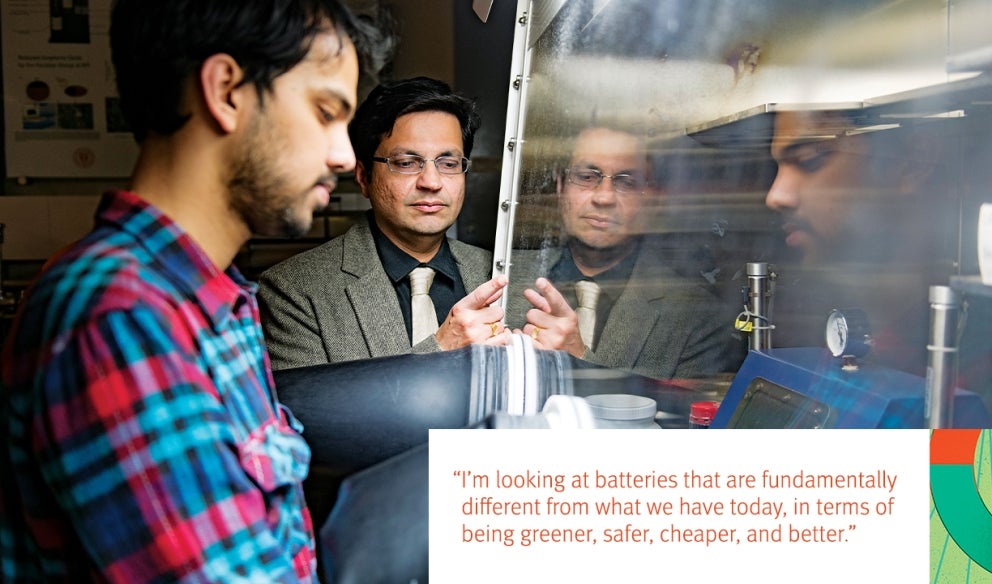
Improving Battery Components
If you were to look inside a battery, you’d find two electrodes — a cathode on one end and an anode on the other.
Inside a lithium-ion battery you’d likely find a cathode made of lithium cobalt oxide and an anode made of graphite. During charging and discharging, lithium ions flow back and forth between the two electrodes through a liquid electrolyte. Much of Koratkar’s work has been focused on methodically improving each of these three components.
For instance, he and his lab explored what would happen if they replaced the traditional graphite-based anode with lithium metal. They wanted to increase efficiency, both in terms of energy density and charging speed — improvements that would allow the battery to store more energy and also charge more quickly. To use lithium metal in this way, researchers first had to solve the persistent challenge of metal layers that accumulate during repeated charge and discharge cycles. The branch-like deposits of metal, known as dendrites, can grow so long that they short
out a battery or even set it on fire.
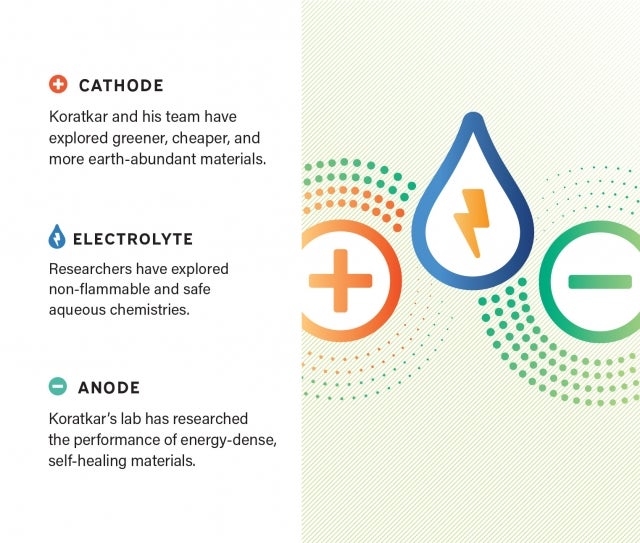
Koratkar and his team discovered how to control the temperature inside the battery so heat would encourage the dendrites to self-heal off the anode. Imagine what happens to a pile of snow after a storm has ended. The wind and sun help move the flakes off the mound, shrinking its size and eventually flattening it out. He compares that process to self-healing. In a similar way, he says, controlled heating within the battery will activate surface diffusion so that metal atoms are moved off the pile that they’ve created, eventually flattening the dendrite out. This revolutionary finding was published in Science and has been successfully replicated by his lab in potassium metal batteries. His approach has now been patented by Rensselaer, and it lays the foundation for more energy-dense, lightweight, and compact batteries.
“This really was a paradigm shift in the way people thought you could use lithium metal in batteries,” Koratkar says. “If you want to change the world, you have to not accept what you’re told and actually verify if there is a better way.” Koratkar’s propensity for tackling challenging questions has resulted in a number of high-impact publications, including in Science, Nature, Nature Communications, Nature Materials, and PNAS. Over the past decade, his research has also been highly cited globally. Earlier this year, he received the William H. Wiley 1866 Distinguished Faculty Award from Rensselaer. “I think citations indicate that our work is having impact. It is of interest to others working in the field, and other researchers are following up on our work — further building on it, and improving it,” Koratkar says. “This tells me that we are working on the cutting edge.”
Looking Back and Ahead
Koratkar often collaborates across disciplines with researchers who have complementary expertise, including those in materials science, mechanical engineering, chemical engineering, and physics. He himself holds a joint appointment in the Department of Materials Science and Engineering and started his career researching nanomaterials.
“Most of the processes that take place inside a battery take place at very small scales,” he says. “I realized that nanomaterials might help a lot in terms of making batteries work better. Presently, we are developing multiscale particles that achieve the optimal blend of micro- and nano-structured properties, which offers exciting possibilities for the future.”
That expertise has played an integral role as Koratkar explores ways to improve lithium-ion batteries, while also imagining alternatives.
“Depending entirely on lithium for our future needs is, I think, very risky,” he says. “I really hope that in five to 10 years, I can present an alternative to lithium that works really well.”
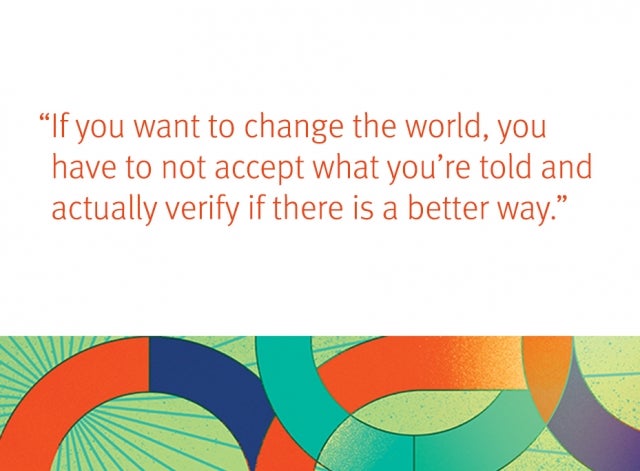
What that battery will look like — or be made of — still isn’t clear, but Koratkar is exploring the potential of more abundant and less expensive materials like sodium, potassium, calcium, magnesium, and zinc. The work his lab has already done provides an important foundation.
For instance, a move away from lithium would also mean that graphite anodes and lithium cobalt oxide-based cathodes would need to be reimagined. His work with transition metal dichalcogenide materials, like vanadium disulfide, and complex oxides, like niobium tungsten oxide, could provide a potential replacement. And, as additional metals are introduced into future batteries, his approach for self-healing metal dendrites will be key.
“I’m looking at batteries that are fundamentally different from what we have today, in terms of being greener, safer, cheaper, and better,” Koratkar says. “I am also looking at multifunctionality, wherein batteries would be flexible, foldable, and could integrate with your structure. Such structural batteries could open the door to the widespread use of batteries in the aerospace industry.”
“There’s a lot of research that needs to go into making existing lithium-ion batteries work better,” he says, “but there are also these other pie-in-the-sky concepts, which may seem far-fetched today, but could revolutionize the way we think about energy storage in the future.”